The Washburn Group
Lignin is the third most abundant biopolymer on Earth (behind cellulose and chitosan). The chemistry of lignin is based on three monolignols, coumaryl alcohol, coniferyl alcohol, and sinapyl alcohol, which are polymerized in situ to form a complex, three-dimensional material.
Purified lignin is obtained through various biomass-processing strategies (usually referred to as “delignification”!), primarily for pulp and paper production, but more recently in cellulosic ethanol production as well as the production of other biomass-derived chemicals. Depending on the source (hardwood, softwood, etc.) and processing conditions, the final product can vary significantly in terms of monomer composition, molecular weight, and the concentration of additional functional groups, such as carboxylates (found in kraft lignin) or sulfonates (found in lignosulfonates). Most commercial lignin forms have molecular weights below 30 kDa, making them organic nanoparticles that can have a diversity of potential uses.
In plants, lignin serves several critical functions, including interfacial binding of cellulose fibers, contributing to mechanical strength, and facilitating water transport. These native functions suggest numerous technological applications of lignin, but lignin finds limited use, primarily as a dispersant in cement and other multi-phase systems. The hypothesis in the Washburn Lab is that grafting synthetic polymers onto a lignin nanoparticle core can leverage these native functions in many applications. Synthesis, characterization, and testing of a broad range of polymer-grafted lignin nanoparticles is a central focus of the Washburn Lab.
References:
- Lignin Biosynthesis and Structure. Ruben Vanholme, Brecht Demedts, Kris Morreel, John Ralph, and Wout Boerjan. Plant Physiology. 2010; 153: 895–905.
- Lignin valorization: improving lignin processing in the biorefinery. A. J. Ragauskas et al. Science. 2014; 344: 1246843.
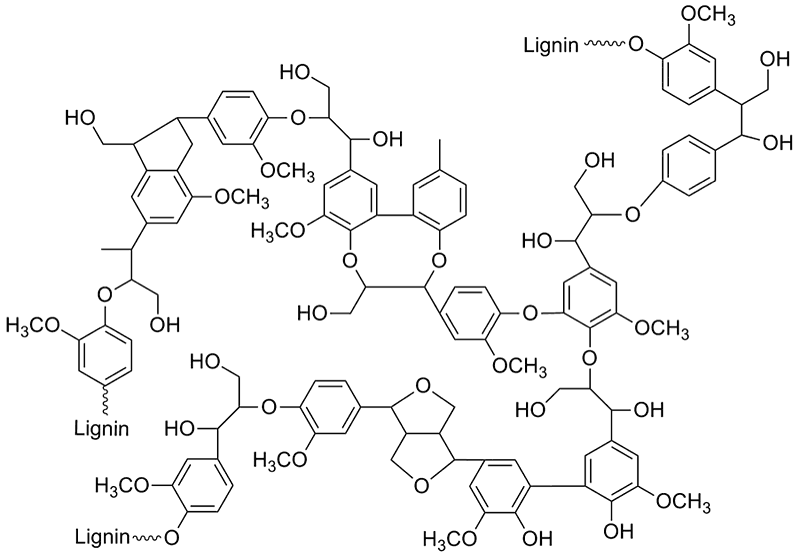
Schematic of native lignin structure showing a diversity of linkages based on ether and alkane groups. The only significantly reactive groups in native lignin are aliphatic and aromatic hydroxyls.
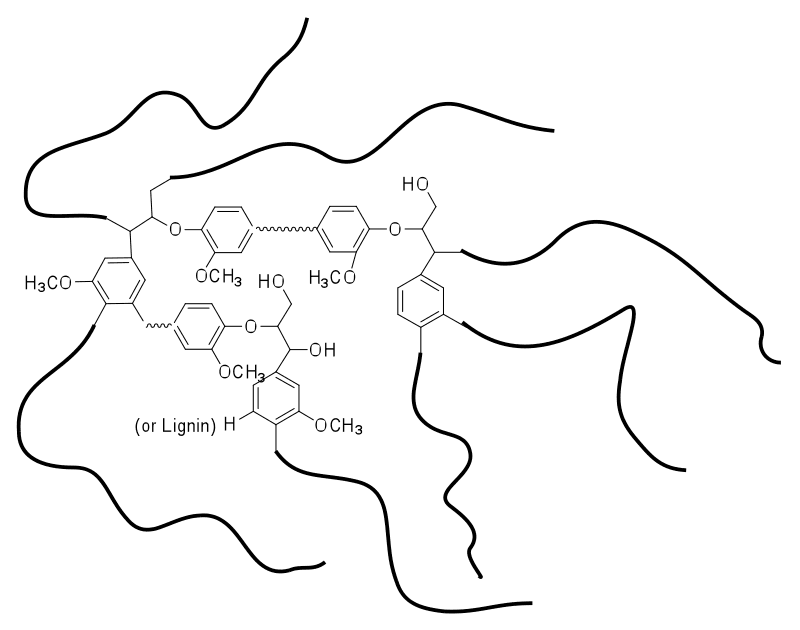
Schematic of a polymer-grafted lignin nanoparticle based on a discrete lignin core with a polymer corona.
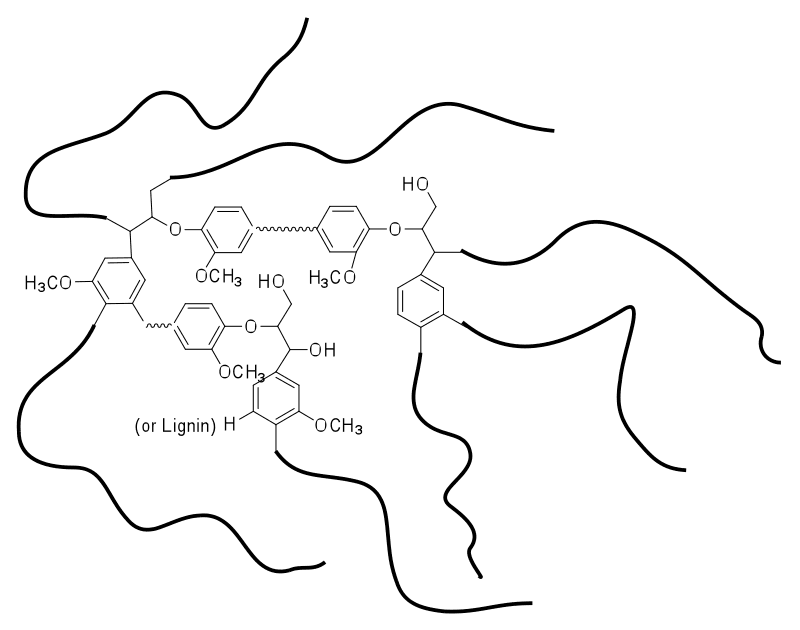
Schematic of a polymer-grafted lignin nanoparticle based on a discrete lignin core with a polymer corona
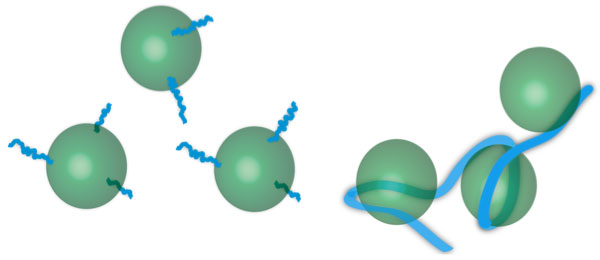
Comparison of polymer grafting architectures based on CRP (left) and FRP (right). The FRP product is crosslinked due to a lack of control over the propagation step, but the CRP product is based on discrete units having a lignin core and a polymer corona. Our hypothesis is that the CRP architecture more effectively leverages the native functions of lignin.
There have been numerous approaches to preparing hybrid materials based on synthetic polymers and lignin, but these generally have relied on free radical polymerization (FRP) chemistries or other conventional polymerization methods, which produce crosslinked products either as nanoscale aggregates or bulk materials. Living polymerizations, such as anionic polymerization or controlled radical polymerization (CRP), offer numerous advantages in preparing polymer-grafted lignin nanoparticles. These include site-specific initiation, control over molecular weight distribution, and control of chain-end functionality.
ATRP and other CRP methods are well suited for grafting a broad range of hydrophilic and thermoplastic polymers from lignin. The group of Prof. John Kadla was the first to report the use of atom transfer radical polymerization (ATRP) in the preparation of lignin grafted with poly(N-isopropylacrylamide), a polymer with unusual temperature-dependent solubility in water. This pointed to a broader range of polymer-grafted lignin nanoparticles that could be prepared using CRP to graft both hydrophilic polymers for the preparation of surfactants and thermoplastic polymers for the preparation of nanocomposites. In the Washburn Lab, we make extensive use of ATRP and related techniques, such as reversible addition-fragmentation chain-transfer (RAFT) polymerization, to explore a broad range of lignin-based nanomaterials.
References:
- Experimental Criteria for Living Polymerizations. R. P. Quirk and B. Lee. Polymer International, 1992; 27: 359–367.
- Preparation of a thermoresponsive lignin-based biomaterial through atom transfer radical polymerization. Y. S. Kim and J. F. Kadla. Biomacromolecules, 2010; 11: 981–988.
- Chemistry of lignin-based materials. H. Chung and N. R. Washburn. Green Materials, 2013; 1: 137–160.
Cement
Cement is the most widely used engineered material on Earth, with over 7 trillion pounds produced globally each year. However, the manufacture of cement contributes more than 5% of total CO2 production, and efforts to reduce this require incorporation of alternative supplementary cementitious materials (ASCMs), such as limestone, clays, or zeolites. The challenge in using ASCMs is they significantly reduce the workability of the cement paste before it has set, making them much harder to use than ordinary Portland cement.
Following the addition of water, cement pastes behave as Bingham fluids, characterized by elastic behaviour up to a yield stress after which the material flows with a characteristic viscosity (toothpaste is a classic example). To improve the workability, a class of polymeric additives known as plasticizers or superplasticizers are used to reduce the yield stress. Lignosulfonates are a widely used class of plasticizer used to improve the workability of cement paste, which are derived from sulfite pulping. However, the leading admixtures for improving workability are poly(carboxylate ether) (PCE) comb copolymers. Our early results show that polymer-grafted lignin nanoparticles could offer performance that rivals PCE.
A standard measure of workability is slump spread measurements, which are simple but actually have a firm theoretical basis in connecting the results to the yield stress of the cement paste. In these tests, wider spreading of the cement paste after it is released from the form is associated with lower yield stresses, scaling as 1/R5 where R is the value for the slump spread.
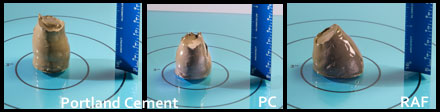
Images of slump spread experiments using a water/cement ratio of 0.42
In our first paper on applications in cement, kraft lignin grafted with polyacrylamide was prepared using controlled radical polymerization (RAFT) and compared with material having the same composition but prepared via free radical polymerization using lignin functionalized with glycidyl methacrylate (GM). Their effects on the workability of cement were compared against a commercial PCE superplasticizer as a function of concentration. The RAFT lignin product performed much better than the FRP product and was competitive with the commercial PCE at high concentrations and was still effective at 10x lower concentration. These results suggest that the dispersant characteristics of lignin can be significantly augmented through controlled polymer grafting.
Improving the properties and reducing the environmental impact of cement are critical issues. It is also an excellent research area for training both science and engineering students in molecular engineering.
References:
- A fifty cent rheometer for yield stress measurement. N. Pashias, D. V. Boger, J. Summers and D. J. Glenister, J Rheol, 1996; 40: 1179–1189.
- Innovation in use and research on cementitious material. K. L. Scrivener and R. J. Kirkpatrick. Cement and Concrete Research. 2008; 38: 128–136.
- Molecular architecture requirements for polymer-grafted lignin superplasticizers. C. Gupta, M. J. Sverdlove, N. R. Washburn. Soft Matter. 2015; 11: 2691–2699.
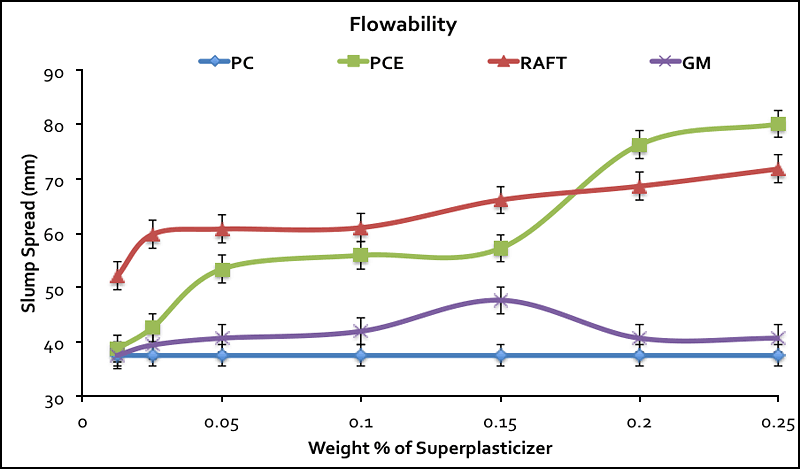
Slump spread values for Portland cement paste as a function of superplasticizer concentration. Neat Portland cement (OPC) was compared with formulations containing kraft lignin grafted with polyacrylamide using controlled radical polymerization (RAFT) and free radical polymerization (FRP) as well as a commercial PCE. Higher slump spread values are associated with improved workability, and the RAFT product showed excellent performance at high concentration and sustained effects even at 10x lower concentration
Surfactants are molecules that compatibilize gases, liquids, and solids. Common classes include foaming agents (gas-liquid), emulsifiers (liquid-liquid), and dispersants (solid-liquid). While classical surfactants are based on polar head groups, which can be anionic, cationic, or non-ionic, with non-polar tails, polymers or nanoparticles often have excellent properties. Different lignin types have been explored for use as foaming agents and emulsifiers, but they generally have low interfacial activities and result in only modest changes in air-water surface tension and are not especially effective at stabilizing oil-water emulsions at neutral pH.
However, it was shown that grafting polymers onto silica nanoparticles significantly enhances their effectiveness in forming Pickering emulsions, and recent studies in the Washburn Lab have shown that similar dramatic improvements in performance are possible with polymer-grafted lignin nanoparticles. Cyclohexane-in-water emulsions with droplet size that depends on polymer grafting density can be formed from kraft lignin grafted with polyacrylamide, and these emulsions are stable against coalescence for months.
Images of cyclohexane-in-water emulsion prepared using kraft lignin grafted with polyacrylamide having degree of polymerization 100 and grafting densities of 2/lignin particle (left) and 16/lignin particle (right).
References:
- Particles as surfactants—similarities and differences. B. P. Binks, Current Opinion in Colloid & Interface Science 2002; 7: 21–41 (2002).
- Interfacial dynamics and rheology of polymer-grafted nanoparticles at air-water and xylene-water interfaces. N. J. Alvarez, S. L. Anna, T. Saigal, R. D. Tilton, and L. M. Walker, Langmuir 2012; 28: 8052–8063.
- Polymer-Grafted Lignin Surfactants Prepared via Reversible Addition—Fragmentation Chain-Transfer Polymerization. C. Gupta and N. R. Washburn, Langmuir 2014; 30: 9303–9312.